Hollow Nickel-Based Alloy Micro-Tubes via Directed Kirkendall Pore Formation
Dr. Alexander Chadwick, *Dr. Dinc Erdeniz, *Dr. Ashley Paz y Puente, and *Aaron Yost
in collaboration with Prof. Ashley Paz y Puente, University of Cincinnati
Metallic structures containing open porosity have low density and high surface area, which are characteristics of interest for a variety of applications from batteries to biomedical implants. In particular, the fabrication of hollow nano- and micro-objects has recently garnered significant attention. Because traditional methods for forming hollow structures, such as tubes, shells, and cages, typically require template removal from the outside in, these geometries are not easily achieved at such small scales. However, by taking advantage of the radial symmetry and spatial confinement of nano- and micro-objects, these hollow structures can be formed using an inside-out approach. The Kirkendall effect, a consequence of the imbalance of diffusivities among atomic species, can result in a supersaturation of vacancies in the material, which often condense to form so-called Kirkendall pores. Typically, these Kirkendall pores are considered detrimental because they deteriorate the mechanical, thermal, and electrical properties of materials, but we focus on the use of the Kirkendall effect as a novel route for the fabrication of β-NiAl(Cr) and shape memory NiTi microtubes.
The current project aims at understanding the wire size effect on the Ni-Ti interdiffusion behavior and Kirkendall pore evolution. Specifically, as the wire diameter (i.e., its volume-to-surface ratio) decreases, we expect a transition from (i) numerous Kirkendall pores in the cross-section (bulk behavior) for large wires, to (ii) a single pore in the cross-section for thinner wires, to (iii) no pores for very thin wires, as vacancies diffuse to the surface instead of forming pores. To this end, we are conducting a systematic study that explores the mechanisms and kinetics of Ni-Ti interdiffusion and Kirkendall pore evolution as a function of wire diameter, ranging from 10 to 200 µm. Pure Ni wires are coated with Ti via pack cementation and subsequently homogenized while capturing the evolution (size, fraction, location) of the various intermediate and final phases (NiTi2, NiTi, Ni3Ti) and the Kirkendall pores. Wires are characterized using both ex situ metallographic techniques and in situ X-ray tomography. Knowledge gained from these experiments will be applied to the fabrication of NiTi (1D) wires, (2D) springs, and (3D) woven scaffolds.
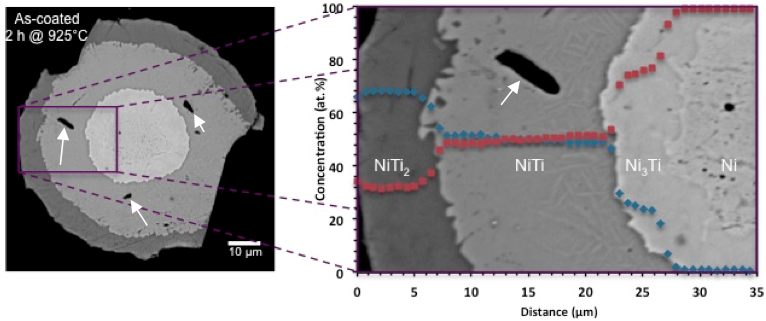
Figure 1. Cross-section of a Ni wire coated with Ti at 925°C for 2 hours. Three distinct shells surrounding a Ni core were identified as NiTi2, NiTi, and Ni3Ti (in order from the surface to the core) via EDS line-scans (Ti: blue, Ni: red). The blocky features (arrows) are embedded TiC particles and missing parts of the wire are due to metallographic preparation.
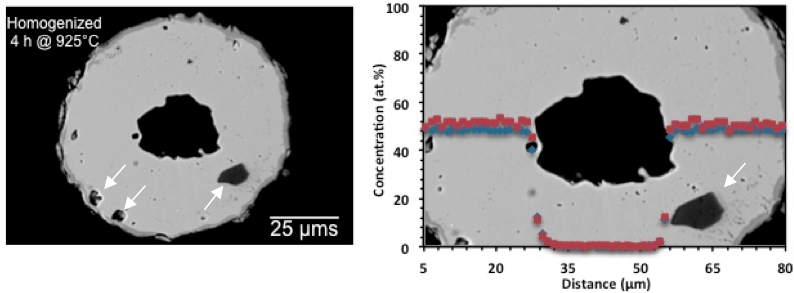
Figure 2. Cross-section of a wire homogenized at 925°C for 4 hours following Ti deposition at 925°C for 2 hours. EDS line-scan data (Ti: blue, Ni: red) confirms full homogenization to near equiatomic B2-NiTi with a near constant composition of Ni-49 at.% Ti. A thin NiTi2 shell remains at the wire surface. A central cavity formed due to the Kirkendall effect. The blocky features (marked with arrows) are TiC particles.
Funding support
- National Science Foundation, DMR--16-11308
- Defense Advanced Research Projects Agency, W91CRB1010004 (Dr. Judah Goldwasser, program manager)
Related Publications
- 1. Paz y Puente, A. P., & Dunand, D. C. (2018). Synthesis of NiTi microtubes via the Kirkendall effect during interdiffusion of Ti-coated Ni wires. Intermetallics, 92, 42-48
- 2. Paz y Puente, A. P., Erdeniz, D., Fife, J. L., & Dunand, D. C. (2016). In situ X-ray tomographic microscopy of Kirkendall pore formation and evolution during homogenization of pack-aluminized Ni–Cr wires. Acta Materialia, 103, 534-546